Study Identifies New Mechanisms of AADC Deficiency Mutations
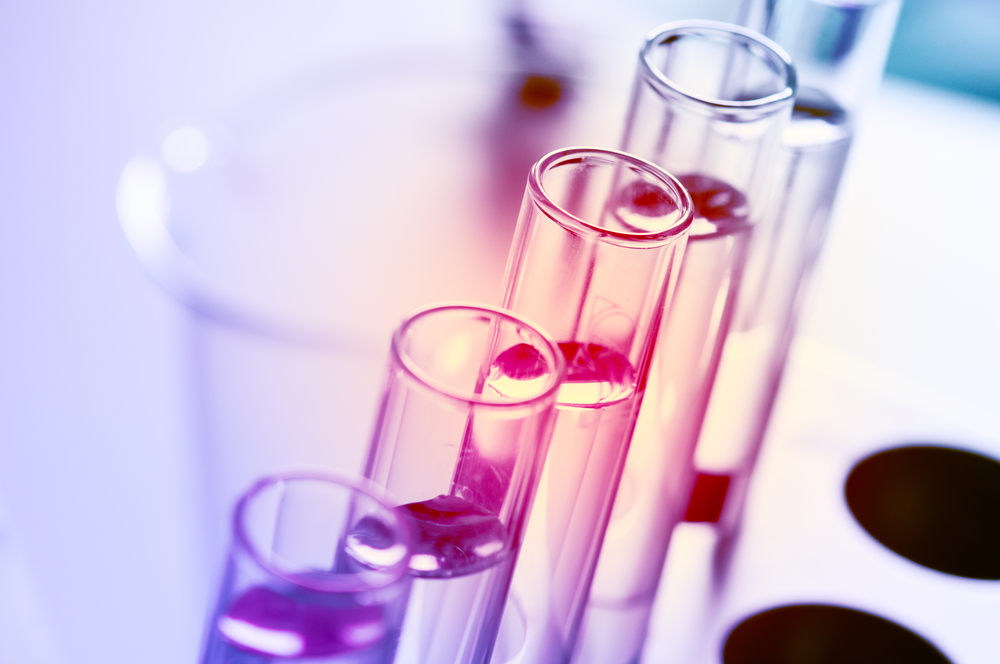
Mutations discovered in the past five years that cause aromatic amino acid decarboxylase (AADC) deficiency affect the functioning of the AADC protein in previously unappreciated ways, namely promoting aggregation and preventing interactions among AADC protein pairs, a molecular study demonstrates.
The new mechanisms identified in the study light the way for more studies about the effects of mutations in AADC deficiency.
The results were published in the Archives of Biochemistry and Biophysics, in the paper “New variants of AADC deficiency expand the knowledge of enzymatic phenotypes.”
AADC deficiency is caused by mutations in the gene that provides instructions for making AADC, a protein important in the synthesis of the neurotransmitters serotonin and dopamine. Since the disease was first discovered, more and more disease-causing mutations have been reported.
All such mutations fundamentally alter the AADC protein, making it unable to carry out its normal function. However, different mutations can affect the protein in different ways, and understanding these differences may be important in understanding individuals’ specific diseases.
Previous studies have found that most reported mutations affect the apo-to-holo transition — that is, they impair AADC’s ability to bind a molecular “helper” called a coenzyme, without which it cannot function properly.
The (non-functional) AADC protein without this coenzyme is called an apoprotein; while the protein with this group is called a holoprotein. That’s why it is called the apo-to-holo transition.
In the new study, researchers characterized 13 recently described AADC deficiency-causing mutations — identified from 2014 to 2019 — looking at how the mutations affected the structure and function of the AADC protein.
These mutations were called H70Y, F77L, V60A, G96R, A110E, L353P, R453C, P210L, L222P, F237S, F251S, W267R, and E283A. The nomenclature refers to the location and exact change in amino acid of the protein sequence (amino acids are the building blocks of proteins). For example, H70Y refers to a mutation that results in a tyrosine (Y) at position 70, where there is a histidine (H) in the healthy protein.
Right away, some of these mutations appeared distinct from those previously reported based on their locations alone. Most mutations associated earlier with AADC deficiency are centrally located in the protein’s sequence — specifically in a part of the protein called loop1 — whereas these were scattered along its length.
The mutant proteins first were produced in bacteria, then purified and subjected to a battery of chemistry tests, such as detailed analysis of the proteins’ catalytic efficiency (i.e., how efficiently they could produce neurotransmitters) and assessments of how light scatters off the protein (which can provide clues about shape and behavior). These analyses were supplemented with computer-based modeling.
“[T]he loop1 variants H70Y and F77L resemble, in terms of catalytic efficiency and of structural conformation, the already studied ones affecting loop1,” the researchers wrote. Essentially, these two mutations were similar, in terms of both location and effect, to the previously-characterized ones in loop1 that affect apo-to-holo transition.
The other variants, however, appeared to impact AADC function through other mechanisms.
Specifically, the mutations P210L, L222P, F237S, F251S, W267R and E283A were all located in another region of the protein, called the large domain. Proteins with these mutations were similar, in terms of catalytic efficiency and structure, to the healthy protein. But all of them were recovered in very low amounts, or weren’t recovered at all, during the process of purifying the proteins for experimentation.
This, combined with analysis of some of the proteins that could be recovered in meaningful quantities, suggested that these mutations might cause the protein to form clumps — or aggregate — in the apoprotein form.
“Only a small fraction is thus able to reach a competent state binding the coenzyme and performing catalysis, while the great part undergoes aggregation in the apo form,” the researchers wrote. They also noted that the extent of this effect, both at the molecular level and symptomatically, varied based on the specific mutation, writing that “[A]lterations in the large domain could give rise to a wide spectrum of disease outputs.”
AADC functions as a dimer – two AADC proteins need to work together to function properly. The remaining mutations — V60A, G96R, A110E, L353P and R453C — were located at the interface where this interaction occurs.
“Since the active sites are at the interface, a defect in this wide part of the protein might affect catalysis and/or coenzyme binding,” researchers noted. Indeed, such mutations tended to result in proteins with very low enzymatic efficiency when tested.
“It is not strange that patients bearing these mutations are severely affected,” the researchers wrote.